The existence of cellulose as the common material of plant cell walls was first recognized by Anselm Payen in 1838. It occurs in almost pure form in cotton fiber and in combination with other materials, such as lignin and hemicelluloses, in wood, plant leaves and stalks, etc. Although generally considered a plant material, cellulose is also produced by some bacteria.
It has been accepted for many years that cellulose is a long chain polymer, made up of repeating units of glucose, a simple sugar. In the early 1900s, cellulose was further characterized by Cross and Bevan. They removed the related plant materials that occur in combination with cellulose by dissolving them in a concentrated sodium hydroxide solution. They designated the undissolved residue as α -cellulose. The soluble materials (designated as β -cellulose and γ -cellulose) were later shown not to be celluloses, but rather, relatively simple sugars and other carbohydrates. The α -cellulose of Cross and Bevan is what is usually meant when the term “cellulose” is used now.
As a carbohydrate, the chemistry of cellulose is primarily the chemistry of alcohols; and it forms many of the common derivatives of alcohols, such as esters, ethers, etc. These derivatives form the basis for much of the industrial technology of cellulose in use today. Cellulose derivatives are used commercially in two ways, as transient intermediates or as permanent products.
Because of the strong hydrogen bonds that occur between cellulose chains, cellulose does not melt or dissolve in common solvents. Thus, it is difficult to convert the short fibers from wood pulp into the continuous filaments needed for artificial silk, an early goal of cellulose chemistry. Several different cellulose derivatives were examined as early routes to artificial silk (see History of Manufactured Fibers), but only two, the acetate and xanthate esters, are of commercial importance for fibers today.
Cellulose acetate is soluble in organic solvents such as acetone and can be spun into fiber or formed into other shapes. Xanthate esters are formed when cellulose is first treated with strong alkali and then exposed to carbon disulfide. Cellulose xanthate is soluble in aqueous alkali; and the resulting solution can be extruded as filaments or films. This is the basis for the viscose process for rayon manufacture.
Recently, technology has been developed to form textile fibers (Lyocell) directly from wood pulp without using a derivative to facilitate dissolution. This technology is based on the ability of amine oxides, particularly N-methylmorpholine N-oxide, to dissolve unsubstituted cellulose.
The Chemistry of Cellulose
Although it took many decades after the identification of cellulose by Payen, cellulose has been shown to be a long chain polymer with repeating units of D-glucose, a simple sugar. In the cellulose chain, the glucose units are in 6-membered rings, called pyranoses. They are joined by single oxygen atoms (acetal linkages) between the C-1 of one pyranose ring and the C-4 of the next ring. Since a molecule of water is lost when an alcohol and a hemiacetal react to form an acetal, the glucose units in the cellulose polymer are referred to as anhydroglucose units.
The spatial arrangement, or stereochemistry, of these acetal linkages is very important. The pyranose rings of the cellulose molecule have all of the groups larger than hydrogen sticking out from the periphery of the rings (equitorial positions). The stereochemistry at carbons 2, 3, 4 and 5 of the glucose molecule are fixed; but when glucose forms a pyranose ring, the hydroxyl at C-4 can approach the carbonyl at C-1 from either side, resulting in two different stereochemistries at C-1. When the hydroxyl group at C-1 is on the same side of the ring as the C-6 carbon, it is said to be in the α configuration (not to be confused with α cellulose, which is not related). In cellulose, the C-1 oxygen is in the opposite, or β configuration (i.e., cellulose is poly[β -1,4-D-anhydroglucopyranose]). This β configuration, with all functional groups in equatorial positions, causes the molecular chain of cellulose to extend in a more-or-less straight line, making it a good fiber-forming polymer. Amylose, a constituent of starch, is a related polymer of glucose, but with the C-1 oxygens in an α configuration. This configuration forces the linkage to the next glucopyranose ring to assume an axial position, and the starch molecules tend to coil, rather than extend. Even though it often has long molecular chains, amylose is not a good fiber-former.
Because of the equatorial positions of the hydroxyls on the cellulose chain, they protrude laterally along the extended molecule. This positioning makes them readily available for hydrogen bonding. These hydrogen bonds cause the chains to group together in highly ordered (crystal-like) structures. Since the chains are usually longer than the crystalline regions, they are thought to pass through several different crystalline regions, with areas of disorder in between (the “fringed-micelle” model). The inter-chain hydrogen bonds in the crystalline regions are strong, giving the resultant fibers good strength and insolubility in most solvents. They also prevent cellulose from melting (i.e., non-thermoplastic). In the less ordered regions, the chains are further apart and more available for hydrogen bonding to other molecules, such as water. Most cellulose structures can absorb large quantities of water (i.e., it is very hygroscopic). Thus, cellulose swells, but does not dissolve, in water.
Reactions of Cellulose
The cellulose molecule contains three different kinds of anhydroglucose units, the reducing end with a free hemi-acetal (or aldehyde) group at C-1, the non-reducing end with a free hydroxyl at C-4, and the internal rings joined at C-1 and C-4. But because of the long chain length, the chemistry of the alcohol groups of the internal units predominates, so long as the chains are not cleaved by the reaction conditions. Unlike simple alcohols, however, cellulose reactions are usually controlled more by steric factors than would be expected on the basis of the inherent reactivity of the different hydroxyl groups. There are potentially three hydroxyls groups available on each anhydroglucose ring, so derivatives are usually characterized in terms of a “degree of substitution” (DS), which is an average for the whole chain and can range between 0 and 3. In most cases, partial reaction to DS < 3 gives products that are essentially block copolymers, where virtually all of the hydroxyls occurring in the less ordered regions may be derivatized, while those in the crystalline regions remain unreacted. Higher degrees of substitution, or reaction conditions which disrupt the crystalline regions, can be used to reduce inter-chain hydrogen bonding and force the chains apart. This can result in a cellulose derivative that is soluble in common solvents, and thus capable of extrusion to form filaments, or other structures.
Esterification
Like simple alcohols, the hydroxyl groups of cellulose can be esterified by reaction with acids or other acylating agents. Nitration with a mixture of nitric and sulfuric acids was used to produce the first man-made fibers (see History of Manufactured Fibers). Acetylation with acetic acid or acetic anhydride produces a variety of different products with properties that depend on the DS.
Etherification
Cellulose ethers can be made using a number of common alkylating agents. Many partially substituted ethers (usually with DS 0.5 – 2.0) are important commercially. These range from simple methyl and ethyl ethers, to more complex materials, such as carboxymethyl celluloses, which are made by reaction of cellulose with chloroacetic acid. Reaction of cellulose with ethylene oxide, or other epoxides, yields hydroxyethyl cellulose, or other hydroxyalkyl derivatives, which can have a number of useful properties, depending on DS and the length of the hydroxyalkyl side chains. Cellulose hydroxyls can also be made to add across activated double bonds, as in the formation of cyanoethyl cellulose from reaction with acrylonitrile.
Acetal Formation
Like simple alcohols, the hydroxyls of cellulose react with aldehydes and hemiacetals to form acetals. Reaction with formaldehyde and formaldehyde derivatives (most commonly urea-formaldehyde resins) is of particular importance for imparting dimensional stability, particularly permanent-press characteristics, to cellulosic textiles such as cottons and rayons.
Hydrolysis
Although cellulose is sufficiently stable toward hydrolysis to allow it to be dyed, finished and laundered, it is susceptible to hydrolysis by acids and, to a lesser extent, by alkalis. Acids attack the acetal linkages, cleaving the 1-4-glycosidic bonds. Since acetals are quite stable toward alkali, hydrolysis at high pH usually requires more vigorous conditions than at low pH. A number of different base-catalyzed reaction pathways are possible and chain cleavage usually results from several concurrent reactions. Cellulose is also degraded by cellulase enzymes.
Oxidative Degradation
Strong oxidizing agents and/or vigorous reaction conditions convert cellulose into CO2 and H2O. Under less vigorous reaction conditions, cellulose is capable of a variety of oxidation reactions, many of which are predictable by analogy to simple alcohols, trans-glycols and acetals. In general, oxidation of cellulosic hydroxyls forms the expected aldehyde and ketone, and carboxyl groups. However, unlike their simple carbonyl analogs, the oxidation products of cellulose (termed oxycelluloses) are significantly less stable in the presence of alkali. Chain and/or ring cleavage occurs by abstraction of the acid hydrogens α to the carbonyl, followed by β -alkoxyelimination.
Non-flaming combustion (or glowing combustion) of cellulose occurs by direct air-oxidation of cellulose at high temperature and produces water, CO and CO2. Although it is difficult to make pure cellulose burn in a non-flaming mode, contaminants, such as alkali metal salts, promote glowing combustion. Flaming combustion is a gas phase oxidation process and, thus, requires prior pyrolytic or thermo-oxidative degradation of the nonvolatile cellulose chains to form flammable, volatile, organic compounds.
Thermal Degradation
A number of different thermal degradation reactions are known to occur with cellulose at different temperatures. Degradation at lower temperatures (as in aging of cellulosic materials) is often predominantly thermo-oxidative and/or hydrolytic. As expected, aging of cellulose is, thus, usually a function of humidity, light, oxygen availability, etc., in addition to temperature. At higher temperatures (>200° C) water is lost, first from that absorbed by the cellulose and then by β -elimination from the cellulose hydroxyls. At still higher temperatures (>250°C), several competing pyrolytic reactions begin to take over. These reactions can be grouped into three basic classifications: the first group occurs at lower temperatures and is similar to the aging reactions. Products are water, CO, CO2 and a carbonaceous char. At higher temperatures, another reaction begins to take over which results in depolymerization of the cellulose chain and formation of anhydroglucose derivatives, volatile organic materials and tars. At still higher temperatures, more-or-less random bond cleavage of cellulose and intermediate decomposition products results in formation of a variety of low molecular weight compounds.
Durable Press Finishing
Fabrics of cellulosic fibers exhibit good dimensional stability in the dry state; but can shrink and/or wrinkle when wet. This occurs because, in the dry state, the cellulose chains are held together by hydrogen bonds between the hydroxyl groups of adjacent chains. In other words, the hydrogen bonds form a cross-linked structure. If a stress, such as twisting or folding, is applied to the dry fabric, the hydrogen bond crosslinks tend to hold the chains in position and cause the fabric to return to their original position when the deforming stress is removed. However, when the fabric is brought into contact with moisture, water molecules can participate in the hydrogen bonding and penetrate between the cellulose chains, effectively breaking up the crosslinked structure. The water molecules act as a plasticizer for cellulose and the chains may move relative to each other. If the fabric becomes wrinkled in the moist state, the chains move to relieve the strain and there is no effective force to return the fabric to its original shape when the stress is removed. Thus, for cellulosic fabrics to exhibit durable press (also termed permanent press or wrinkle-resistant) characteristics, it is necessary to form crosslinks which are not easily broken by water. This is usually done with formaldehyde or formaldehyde derivatives, such as urea-formaldehyde resins.
Most commercial durable press treatments in use today utilize N-methylol compounds, such as dimethyloldihydroxyethyleneurea (DMDHEU). In the presence of heat and Lewis acid catalysts, such as ZnCl2 or MgCl2, these N-methylol compounds react readily with the hydroxyl groups of adjacent cellulose chains, forming
the desired crosslinks. These crosslinks are quite stable to laundering and allow the fabric to be put through machine washing without wrinkling, or losing desirable pleats and/or creases which were set in prior to crosslinking. Unfortunately, the heat and acid used to cause the crosslinking reaction can cause weakening of the fabric and shortened wear life. To get around this problem, the polyester fibers are often blended with cotton or rayon to give extra strength to the durable press fabric.
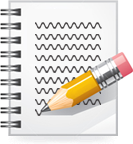